Introduction
Vitamin D (VD) is a steroid hormone and regulates calcium and bone metabolism. VD also plays a role in several other biochemical mechanisms within the body [1, 2]. The mechanism of action of the active form of VD is mediated by the VD receptor (VDR). VDRs are found in cardiomyocytes [3, 4].
Serum VD deficiency is a common condition in population-wide studies. Epidemiologic studies have shown that VD deficiency was associated with chronic diseases such as cancer, type 2 diabetes, autoimmune diseases and cardiovascular disease (hypertension, coronary artery disease, peripheral artery disease, heart failure and stroke) [5, 6]. Thus, VD deficiency can be a cardiovascular risk factor and associated with cardiovascular morbidity and mortality [7, 8].
VD has been related to some pathological changes in the myocardium in previous studies [9–11]. However, the effect of VD deficiency on the deformation parameters of the myocardium is very limited. Conventional echocardiographic parameters such as LV ejection fraction (LVEF) are not sensitive enough to detect subclinical cardiac systolic dysfunction. The 2D STE is a relatively novel and sensitive echocardiographic modality that can assess regional and/or globally subtle LV myocardial impairment with strain and strain rate measurements [12]. The aim of the present study was to evaluate the association of VD deficiency and longitudinal deformation of the LV myocardium measured by 2D STE in diabetic and non-diabetic patients without significant coronary artery disease.
Material and methods
The present study was an observational cross-sectional study, including 140 patients who were admitted to the Istanbul Faculty of Medicine, Department of Cardiology with symptoms of stable ischemic heart disease in the period 2014–2019. The patients underwent coronary angiography and none of them had obstructive coronary artery disease. Obstructive coronary artery disease was defined by the presence of a plaque with 50% or greater stenosis on epicardial coronary arteries. Age, sex, body mass index (BMI), coronary artery disease risk factors (hypertension, diabetes mellitus, dyslipidemia, smoking) and the usage of cardio-protective drugs were recorded. Blood samples were obtained for measurement of 25(OH)D3. All patients were evaluated with conventional echocardiographic parameters, tissue Doppler echocardiography, and 2D STE to assess left ventricular function.
The study was approved by the local ethics committee. All patients gave informed consent. Exclusion criteria were previous myocardial infarction, cardiomyopathies, EF less than 50%, severe valvular disease, chronic renal and liver disease, active malignancies, sustained supraventricular or ventricular arrhythmias during angiography, and the usage of VD replacement therapy.
Laboratory method
To avoid seasonal variations, all blood samples were collected during winter (November–February). Blood samples were stored at –70°C within 30 min of collection. Serum 25 (OH)D3 concentrations were measured using an automated Vitamin D2-D3 HPLC Analyzer (Zivak Technologies 25-OH Vitamin D2-D3 HPLC analysis kit). The intra- and inter-assay coefficients of variation were 7% and 11%, respectively. Patients were categorized into two groups as vitamin D deficient < 20 ng/ml and vitamin D sufficient ≥ 20 ng/dl) according to Endocrine Society guidelines [13].
Echocardiography
One experienced observer performed echocardiography on all subjects with an iE33xMATRIX ultrasound system (Philips Medical Systems, Andover, Massachusetts) with an X5-1 (1–5 MHz) transducer using second harmonic imaging. Conventional echocardiographic measurements were performed in accordance with the recommendations of the American Society of Echocardiography guidelines [14]. Measurements were obtained with parasternal and apical views. The LV dimensions used an average of five M-mode measurements and included diastolic and systolic diameters, diastolic thickness of the interventricular septum and diastolic posterior wall thickness. Systolic function of the LV was determined as fractional shortening and EF was determined via two-dimensional M-mode.
LV diastolic function was assessed using pulsed Doppler measurements of filling in the apical four-chamber view. The Doppler beam was aligned perpendicularly to the mitral annulus plane and the sample volume was placed between the tips of the mitral leaflets. Five consecutive beats of mitral valve inflow were collected to calculate peak early diastolic filling (E wave) and late diastolic filling (A wave) velocities, the E/A ratio, and deceleration time (DT) of the E wave. Early diastolic (e′) and late diastolic (a′) velocities were measured in the septal and lateral wall using a 5 mm circular sample volume placed in the left ventricular myocardial just proximal to the mitral annular level and the mean of septal and lateral e′-a′ velocities accepted. The isovolumic relaxation time (IVRT), isovolumic contraction time (IVCT) and ejection time (ET) values were determined [15]. Myocardial performance index (MPI) is an index of global ventricular function defined as the sum of IVCT and IVRT divided by ET [16]. All size and mass indices were indexed to body surface area.
2 D Speckle Tracking Strain Imaging
Strain was defined as the instantaneous local trace lengthening or shortening and strain rate was the rate of lengthening or shortening [16]. Speckle tracking strain imaging showed three consecutive cardiac cycles from the apical 4-, 3- (long axis), and 2-chamber views. These were obtained at 42–56 frames per second and then stored. The aCMQ option of the QLAB 10.8.5 software was used for deformation analysis. First, for each view, the operator placed three points (two points at the base of the LV and one point at the apex) at the end of diastole. The endocardial and epicardial borders were then automatically traced by the software. Adjustments were made by the operator if required. Systolic strain and strain rate values were calculated at aortic valve closure time by averaging the values of all segments for assessment of LV global longitudinal systolic function [17].
Each wall of the LV was segmented into three (base, mid and apical) equal parts automatically and 18 segmental strain curves were obtained (Figure 1) to give the so-called bull’s-eye plots (Figure 2). Segments with poor tracking despite manual readjustments of the region of interest were excluded. Healthy individuals had an average peak systolic LV longitudinal strain as assessed by speckle tracking from –18 to –20% [17, 18]. The global longitudinal strain (GLS) was determined to be reduced or normal (Figures 1, 2).
Statistical analysis
Statistical analysis was performed with SPSS 19.0 for Windows (IBM Corp. since v. 19.0, Chicago, IL, USA). Continuous data are expressed as the mean ± SD, categorical data are expressed as percentages. The χ2-test was used to assess differences between categorical variables among the groups. The relationships among parameters were assessed using Pearson correlation analysis. Student’s t-test compared unpaired samples. Logistic regression analyses of strain and strain rate by vitamin D insufficiency and traditional cardiac risk factors (age, sex, smoking, DM, HT, and HL) were evaluated among patients. A p-value < 0.05 was considered statistically significant.
Results
Clinical characteristics
We enrolled 140 patients with 80 diabetics and 60 non-diabetics. The gender ratio and cardiovascular risk factors were similar in both groups. Diabetic patients had a lower glomerular filtration rate (GFR) (77.96 ±19.06; 94.08 ±26.10; p < 0.001) and a higher BMI (25.99 ±1.77; 23.89 ±2.8; p < 0.001). Cardiovascular drug usage such as angiotensin converting enzyme inhibitors (p < 0.001), β-blocker (p = 0.031) and statins (p = 0.005) were higher in the diabetic group (Table I). Insulin therapy was prescribed to 38 (48%) patients and these patients had a higher HbA1c level (8.1 ±1.1 vs. 7.0 ±1.1%, p < 0.01) than patients not taking insulin therapy.
Table I
Clinical characteristics of diabetic and non-diabetic patients
The average 25(OH)D3 level for all groups was 16.2 ng/ml (range: 1.6–48, 8 mg/dl). The prevalence of VD deficiency in the diabetic group was 68% and 63% in the non-diabetic group (p = 0.456). Patients with VD deficiency were more likely to be female. There was no significant difference in age or cardiovascular risk factors between the two groups (Table II).
Table II
Clinical characteristics of patients with and without vitamin D (VD) deficiency
Echocardiographic parameters
Conventional echocardiographic parameters of LV systolic and diastolic function in diabetic and non-diabetic patients are presented in Table III. There were no significant differences in LV volumes, EF, wall thickness or LV mass index. In the diabetic group, diastolic function parameters were significantly impaired. The MPI was significantly increased in the diabetic group (p < 0.001). The LV GLS was significantly impaired in diabetic patients versus the non-diabetic group (t = 2.353, p = 0.020). The LV global longitudinal strain rate (GLSR) was significantly impaired in diabetics versus non-diabetics (t = 2.461, p = 0.030).
Table III
Echocardiographic parameters of diabetic and non-diabetic patients
[i] LV – left ventricular, EF – ejection fraction, IVST – interventricular septum thickness, PWT – posterior wall thickness, LVEDV – left ventricular end diastolic volume, LVESV – left ventricular end systolic volume, LVMI – left ventricular mass index, E – early diastolic velocity of mitral valve inflow, A – late diastolic velocity of mitral valve inflow, E′ – early diastolic velocity at basal mitral annulus, A′ – late diastolic velocity at basal mitral annulus, DT – deceleration time, GLS – global longitudinal strain, SR – strain rate, MPI – myocardial performance index, IVRT – isovolumetric relaxation time.
Conventional echocardiographic parameters of LV systolic and diastolic function in patients with and without VD deficiency are presented in Table IV. There were no significant differences in LV volumes, EF, wall thickness, LV mass index, diastolic grade, E-E’ velocity, DT, IVRT or MPI in patients with and without VD deficiency. The LV GLS was significantly impaired in patients with VD deficiency (p < 0.001) versus those without VD deficiency. The LV GLSR was significantly impaired in patients with VD deficiency (p = 0.003).
Table IV
Echocardiographic parameters of patients with and without vitamin D deficiency
[i] LV – left ventricular, EF – ejection fraction, IVST – interventricular septum thickness, PWT – posterior wall thickness, LVEDV – left ventricular end diastolic volume, LVESV – left ventricular end systolic volume, LVMI – left ventricular mass index, E – early diastolic velocity of mitral valve inflow, A – late diastolic velocity of mitral valve inflow, E′ – early diastolic velocity at basal mitral annulus, A’ – late diastolic velocity at basal mitral annulus, DT – deceleration time, GLS – global longitudinal strain, SR – strain rate, MPI – myocardial performance index, IVRT – isovolumetric relaxation time.
Among the diabetic group, VD deficiency is significantly associated with impaired GLS (p < 0.001) and GLSR (p = 0.004). However, there was no significant effect of VD on diastolic function parameters or MPI in diabetics.
Among the non-diabetic group, VD deficiency was associated with impaired GLS (p = 0.049). GLSR was not significantly different between those with and without VD deficiency (p = 0.060). Again, there was no significant effect of VD on diastolic function parameters or MPI in non-diabetics. The correlation of VD and LV GLS is presented in Figure 3.
Figure 3
Correlation of VD levels and left ventricle global longitudinal strain in patients with vitamin D deficiency and without vitamin D deficiency
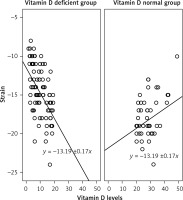
GLS was negatively associated with the level of 25(OH)D3 in the VD deficient group (r = –0.52623, p < 0.001). Conversely, GLS was positively associated with the level of 25(OH)D3 in the VD sufficient group (r = 0.286465, p = 0.048). Logistic regression analysis demonstrated that LV GLS was independently associated with VD deficiency, hypertension, triglyceride levels, age and BMI. GLS was positively associated with age (r = 0.22, p = 0.010), BMI (r = 0.26, p = 0.002) and triglyceride levels (r = 0.19, p = 0.020). There was no correlation between GLS and insulin therapy (t = 1.663, p = 0.098) or HbA1c levels (r = 0.041, p = 0.714) in the diabetic group.
Discussion
It is estimated that most people worldwide are vitamin D deficient [19]. Recent studies demonstrated that VD deficiency was a significant risk factor for cardiovascular disease and associated with cardiovascular mortality [20, 21]. The 25(OH)D3 levels are also associated with important cardiovascular disease risk factors such as diabetes mellitus, obesity, increased levels of triglycerides and hypertension [22–24].
The mechanism underlying the role of VD in the prevention of heart disease remains incompletely understood. However, the proposed mechanisms involve the presence of VDR in vascular smooth cells, endothelial cells and cardiomyocytes. VD can also affect cardiac function by altering parathormon (PTH) and serum calcium levels, regulation of hormone secretion, regulation of the renin–angiotensin–aldosterone (RAA) system, and regulation of blood pressure effects [25]. Activated 1,25(OH)2 D suppresses renin gene expression in vitro and regulates the growth and proliferation of vascular smooth muscle cells and cardiomyocytes [25, 26]. VD induces pathological changes in the myocardium that are similar to pathological changes induced by diabetes [27]. Thus, these two conditions may lead to defects in myocardial deformation that are detectable by 2DSTE. The common pathways that cause myocardial damage include RAA activation, apoptosis and steatosis, leading to myocardial fibrosis, hypertrophy, lipid accumulation in the myocardium [27, 28], matrix metalloproteinase activation [28] and oxidative stress [29]. These features are seen in both diabetes and VD deficiency.
Collectively, these mechanisms may explain the relationship between VD deficiency and myocardial dysfunction although further evaluation is required.
In this study, we found no significant association between VD status and LVEF and LV diastolic function parameters (E, E′, DT, IVRT, MPI). There was a significant association between VD deficiency and impaired GLS and GLSR in both diabetic and non-diabetic groups versus the control group. Notably, this association was independent of the broad spectrum of potential confounders including classical risk factors. That means that VD deficiency was independently associated with impaired LV GLS and GLSR and it is reasonable to think that VD deficiency can actually lead to myocardial dysfunction independently. VD likely affects systolic longitudinal motions initially before diastolic functions in the LV.
We also determined that 2D STE was better than conventional echocardiographic parameters LVEF and fractional shortening (LVFS) in detecting myocardial function in patients with VD deficiency. To our knowledge, this is the first study to investigate the association between VD deficiency and impaired subclinical myocardial dysfunction in diabetic and non-diabetic patients with normal coronary arteries [30].
Chen et al. studied diabetic patients with and without VD deficiency [31]. In this study, the patients had no coronary artery disease. They reported that diabetic patients with VD deficiency have impaired GLS versus diabetics with normal VD levels. In contrast, we included non-diabetic patients and the effect of VD on myocardial function and deformation parameters was also demonstrated. Also, the absence of coronary artery disease was confirmed by selective coronary artery angiography in diabetic and non-diabetic patients.
Sunbul et al. [32] studied 50 non-diabetic patients with VD deficiency who had no coronary artery disease. The evaluation of the coronary artery disease was based only on the patients’ history. All patients were treated with VD analogs. They noted an improvement in systolic longitudinal, radial and circumferential strains of the LV after therapy. These two studies support our study but there are some differences in study designs.
Another surprising result of our study was the significant negative correlation between VD and GLS in the group with VD deficiency (r = –0.52623, p < 0.001); a statistically significant positive correlation was found between VD and GLS in the group without VD deficiency (r = 0.286465, p = 0.048). The range of VD should be 20–40 ng/dl for optimum myocardial longitudinal systolic strain values. This result suggests that myocardial function is suppressed when the VD exceeds a critical value and exaggerated replacement may negatively affect the myocardium.
Hypervitaminosis D occurs when pharmacologic doses of VD are consumed for prolonged periods of time or from a single megadose [33]. No study has yet investigated the effect of high dose VD on myocardial function. The toxic effects of high dose VD have been demonstrated in previous studies. One study examined the effects of VD and VD analogs and showed that exposure of human bone cells to VD in culture increases the cells’ mRNA expression and activity and thus increases oxygen radical production. These authors suggested that potential cell damage is caused by an increase in lipid oxidation in the cell membrane or intracellular compartments due to oxidative stress [34]. Another study showed that VD exposure at high supraphysiological concentrations caused toxic effects on skeletal muscle cells that inhibited myogenesis in culture medium [35].
A previous study used scanning electron microscopy to examine the hearts of Wistar rats treated with a high dose of VD. Multifocal cardionecrosis was detected macroscopically in 70% of the rats on the 7th day when the rats were in the acute phase of intoxication. To explain VD-induced cardionecrosis, they suggested that calcium-activated neutral proteinases may have contributed to the structural alteration of the extracellular matrix components [36, 37]. Nevertheless, the present study is the first to demonstrate that VD toxicity leads to myocardial damage.
This study has a relatively small sample size and is cross-sectional. Therefore, prospective investigations – possibly with randomization to VD supplementation – are warranted. Furthermore, serum VD levels may change throughout the day and across seasons. Thus, a single measurement may not reflect the actual VD status.
In conclusion, this study demonstrated that lower VD levels were significantly associated with impaired myocardial deformation parameters in diabetic and non-diabetic patients with normal coronary arteries and preserved EF. Lower VD levels may play a role in the pathogenesis of myocardial dysfunction. VD deficiency is a pandemic disease but is rarely diagnosed and treated by physicians although it is easy and affordable to treat. The range of vitamin D required for optimal myocardial longitudinal systolic strain values is 20–40 ng/dl, and unnecessary replacement should be avoided.