Introduction
Various types of haematopoietic cells, such as Th17 cells, γδ T cells, natural killer (NK) T cells, macrophages, dendritic cells, neutrophils, and mast cells, can produce interleukin-17 (IL-17) in response to pro-inflammatory cytokines [1]. Th17 cells may directly derive from naïve T cells that are generated in the thymus and are therefore called natural Th17 (nTh17) cells, but they can also be induced in the periphery from naïve cells, or they can result from the conversion of other cell types [2]. The IL-17 family of cytokines contains six isoforms, from A to F; however, Th17 cells are able to produce only IL-17A and IL-17F, both of which are pro-inflammatory cytokines. There is evidence that IL-17A and/or IL-17F are/is responsible for the development of inflammation in many disorders, especially in autoimmune diseases like rheumatoid arthritis (RA), psoriasis, juvenile idiopathic arthritis (JIA), Crohn’s disease, and many others [3]. Th17 cells show a high degree of plasticity thereby exhibiting trans-differentiation to Th1 or Treg cells, as well as TR1, Th2, or TFH cells. This potential endows them with multiple and opposing functions, therefore allowing them to elicit qualitatively distinct responses depending on different micro-environments [4]. In humans, IL-17/interferon-γ (IFN-γ) dual-producing T cells are described in several inflammatory autoimmune diseases such as Crohn’s disease [5], rheumatoid arthritis [6], multiple sclerosis, and atherosclerosis [7, 8]. Plasticity between Th17 and Treg cells has also been reported where Foxp3+IL-17+CD4+ cells were detected in psoriatic lesions [9]. These cells are also detected in the synovia of patients with active rheumatoid arthritis [10]. The interrelation between Th17 and Treg populations is probably very important in the pathogenesis of autoimmune and inflammatory diseases because deviation of critical balance in favour of Th17 cells significantly enhances the severity of disease.
Atherosclerosis, a chronic inflammatory disease of the artery walls, affects human beings from all social, ethnic, and economic backgrounds [11]. Atherosclerotic lesions contain monocytes, macrophages, smooth muscle cells (SMCs), and T lymphocytes [12]. T cells constitute ≈10% of all cells in human plaques, of which 70% are described to be CD4+ and the remaining are largely CD8+ [13]. Tregs and Th17 cells are the two main CD4+ T cell subsets that have important roles in the pathogenesis of atherosclerosis. Th17 cells [8] and IL-17+δγ T cells [14] have recently been detected within human and mouse atherosclerotic vessels. An increase in the circulating levels of Th17 cells has been observed in patients with acute chronic syndrome (ACS) compared with patients with stable angina and healthy controls, and the number and function of Tregs are reported to be reduced as well [15, 16]. The Treg/Th17 ratio and Treg frequency are reported to be negatively correlated with levels of serum oxidised low-density lipoprotein (Ox-LDL), high-sensitivity C-reactive protein (hsCRP), lipoprotein (a) (Lp[a]), and MB iso-enzyme of creatine kinase (CK-MB), while Th17 frequency positively correlated with levels of these biomarkers [17]. While the extent of the role that Th17 plays in the progression of atherosclerosis is still controversial, we and others found an increased frequency of Th17 cells in patients with atherosclerosis [18, 19]. In this regard, deficiency or depletion of IL-17, the signature Th17 cytokine, or its receptor is reported to be atheroprotective [14, 20, 21]. IL-17 maintains plaque stability by inducing proliferation of SMCs and collagen content in atherosclerotic plaques [22]. In addition, IL-17 can down-regulate the expression of vascular cell adhesion molecule (VCAM)-1 in endothelial cells and prevent monocyte adherence; it can also prevent T cell infiltration into plaques [23]. On the other hand, IL-17 can induce the release of chemokines such as CXCL1, CXCL2, CXCL8, and CXCL10, and the chemokines can then recruit neutrophils and monocytes to the atherosclerotic lesion [24, 25]. IL-17 can also stimulate macrophages to produce inflammatory cytokines, such as IL-6, TNF-α, and IL-1β [26–28]. Moreover, IL-17 can promote matrix metalloproteinase (MMP) production (MMP-1, 3, 9, and 13) in fibroblasts, endothelial cells, and epithelial cells [29, 30]. IL-17 induces apoptosis of vascular endothelial cells by activating caspase-3 and caspase-9 and by increasing the Bax/Bcl-2 ratio [31].
In addition to Th17 cells, Tregs which are found in atherosclerotic lesions may play a protective role in the atherosclerosis [32]. Tregs are divided into two main categories: induced Tregs (iTregs) and natural Tregs (nTregs) [33]. nTregs have two subtypes known as resting (CD4+CD25+Foxp3+CD45RA+) and effector (CD4+CD25hiFoxp3hiCD45RO+) Tregs [34]. iTregs, on the other hand, are generated in the periphery from CD4+CD25− T cells and seed for Tr1 and Th3 subsets [33]. It is reported that patients with coronary artery disease (CAD) have reduced peripheral Treg-cell numbers, determined as CD4+CD25+Foxp3+, CD4+CD25hi, or CD4+TGF-β+Th3 cells [35]. In addition to preventing the accumulation of inflammatory cells and their cytokines, increasing conversion of M1 to M2 macrophages [36], increasing secretion of anti-inflammatory cytokines, and inhibition of B cell activation, Tregs may affect blood cholesterol levels. Accordingly, previous studies showed that expansion of Tregs stabilises atherosclerotic lesions, and adenovirus-mediated IL-10 gene transfer in Ldlr–/– mice reduces atherogenesis [37, 38]. Moreover, PEGrIL-10 enhances cholesterol uptake by Kupffer cells concomitant with reduction of all forms of plasma lipids in Ldlr–/– mice.
Previous information suggests that the plasticity of CD4+ T cells may be a game changer in favour of atherosclerosis progression [39, 40]. The recruitment of CD4+ T cells to the lesion and chronic exposure to inflammatory cytokines may provide an environment in which Tregs express IL-17 and exhibit deficient anti-inflammatory response. In a previous report, we showed that both resting and activated Tregs decrease in atherosclerosis [41]. The decrease in CD4+CD45RO+CD25hiFoxp3hi in our patients was simultaneous with an increase in CD4+CD45RO+CD25+Foxp3- effector/memory T cells [41]. In the current study, we investigated the possibility of IL-17 production by Tregs (Foxp3+) in patients with atherosclerosis, in comparison with that of individuals with normal/insignificant arterial lesion. Our results indicated that the frequencies of IL-17+Foxp3+ and IL-17+Foxp3- CD4+ T cells were higher in patients than in controls before and after stimulation. We also found that after stimulation with PMA/ION, the percentage of CD4+CD45RO+IL-17-Foxp3+ T cells (activated T regulatory – aTregs) increased in controls compared with patients (p = 0.0006). But the frequencies of CD4+CD45RO-IL-17+Foxp3- T cells (Th17) and CD4+CD45RO+IL-17+Foxp3- T cells were higher in patients than in controls (p = 0.004 and p = 0.0001, respectively). In the patient group, the frequency of effector/memory T cells remained higher than in controls after stimulation (p = 0.004). The percentage of nTregs was increased in controls compared with patients after stimulation with PMA/ION (p = 0.04).
Material and methods
Subjects
This study was approved by the Ethics Committee of Shiraz University of Medical Science (SUMS). The participants were informed about the aim of this study as well as the safety and security measures, and then the written consent was obtained. After detection and confirmation of atherosclerosis by coronary angiography, 15 ml of heparinised blood was obtained from each of the 10 non-diabetic patients (5 men and 5 women aged 60.4 ±12.91 years) who were diagnosed with coronary artery disease. The control group consisted of 7 non-diabetic, non-smoking individuals (3 men and 4 women aged 50.85 ±11.75 years) with normal coronary angiography/insignificant CAD. Peripheral blood mononuclear cells (PBMCs) were separated from blood by density gradient centrifugation on Ficoll. Flow cytometry experiments were performed both without stimulation and after stimulation with PMA (50 ng/ml) and ionomycin (250 ng/ml), using fluorescent antibodies against CD4, CD45RO, IL-17, and Foxp3 markers, for detection of CD4, CD45RO, IL-17, and Foxp3 expression.
Isolation of peripheral blood mononuclear cells
PBMCs were isolated by density gradient centrifugation (Ficoll-Paque PLUS, Inno-train, Germany) and cryopreserved in 10% dimethyl sulfoxide (DMSO; Sigma-Aldrich) in foetal bovine serum (FBS Biosera, UK).
Treg subset detection by flowcytometry
For enumeration of Th17 and Treg cells, PBMCs (1 × 106 cells) were washed and divided in two tubes. The cells in one tube were kept un-stimulated and the cells in the second tube were stimulated. Stimulation was performed with phorbol myristate acetate (PMA, 50 ng/ml, Sigma) plus ionomycin (ION, 250 ng/ml, Sigma) at 37°C and 5% CO2. Golgi-stop was added to both tubes after 1 h, and the cells were incubated for another 18 h at 37°C and 5% CO2. Then the cells were washed and stained using conjugated antibodies: anti-CD45RO-FITC (BD Pharmingen), anti-CD4-PerCP (BD Pharmingen), anti-IL-17-Alexa fluor 647 (BD Pharmingen), and anti-Foxp3-PE (BD Pharmingen) and were incubated at 4°C for 30 min. For intracellular staining of Foxp3 and IL-17 molecules, the cells were fixed and permeabilised by Foxp3 buffer set (BD, USA) before adding the conjugated Foxp3 and IL-17 antibodies. The cells were subsequently washed and resuspended in PBS containing 10% FBS. For each sample, 1 × 105 cells were acquired by FACScalibur flowcytometer. Live lymphocytes were gated on forward and side scatter, and flowcytometry analysis was carried out by FlowJo software (version 7.6.2).
Results
Frequencies of IL-17- and Foxp3-expressing CD4+ T cells
After gating on live CD4+ T cells we evaluated the T helper subsets in patients and controls based on the expression of IL-17 and Foxp3 in non-stimulated and stimulated conditions (Figure 1 A). Interestingly, we found significantly higher frequencies of IL-17+Foxp3+ and IL-17+Foxp3- cells both in the presence (p = 0.0027 and p = 0.0013) and absence (p = 0.0031 and p = 0.033) of PMA /ION stimulation in patients than in controls (Figure 1 B). This was accompanied by a decrease in the IL-17-Foxp3+ and IL-17-Foxp3- populations in patients.
Figure 1
Frequencies of IL-17 and Foxp3 in the CD4+ T-cell population. A – Representative dot plots illustrating production of IL-17 and Foxp3 in the CD4+ T cells in a control individual and in a patient with atherosclerosis in non-stimulated and stimulated conditions. B – Frequencies of IL-17+Foxp3+ and IL-17+Foxp3- CD4+ lymphocytes were higher in patients both in non-stimulated and in PMA/ionomycin stimulated conditions. The cells were gated on CD4+ population after gating on live lymphocytes, and then the frequencies of IL-17 and Foxp3 subsets were determined. Two of the control samples were lost after stimulation and are not included in the results
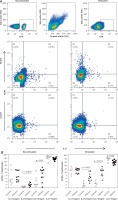
Treg/Th17 ratio in patients with atherosclerosis and controls in non-stimulated and stimulated conditions
We observed an imbalance in Treg (CD4+- Foxp3+)/Th17 (CD4+IL-17+) frequencies in patients with atherosclerosis disease. Our result showed a Treg/Th17 decrease in patients compared with controls in non-stimulated and PMA/ION stimulated conditions (Table I).
Frequencies of IL-17- and Foxp3-expressing CD4+CD45RO+ T cells
As shown in Figures 2 A and B, the frequency of CD4+CD45RO+IL-17+ Foxp3+ (aTreg) cells was higher in patients compared with controls before and after stimulation with PMA/ION (p = 0.0136 and p = 0.0027). After stimulation, the frequency of CD4+CD45RO+IL-17+Foxp3+ lymphocytes increased to a greater extent in patients (p < 0.0001) compared to controls (p = 0.149).
Figure 2
The gating strategy for defining IL-17- and Foxp3-expressing memory subpopulations. The cells were gated on live lymphocytes, and then based on the expression of CD4 and CD45RO the memory/effector T populations were defined. The frequencies of IL-17 and Foxp3 subsets were determined afterwards in each subset
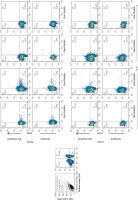
The frequency of the CD4+CD45RO+IL-17+ Foxp3– effector/memory T-cell subset was higher in patients than controls, and it increased after stimulation (p = 0.0250, p = 0.0007). The frequency of the CD4+CD45RO+IL-17-Foxp3- T cells was higher in controls after stimulation with PMA/ION (p = 0.04).
Frequencies of IL-17- and Foxp3-expressing CD4+CD45RO- T cells
The frequencies of CD4+CD45RO-IL-17- Foxp3+ (nTregs) did not show any difference between patients and controls (Figures 2 C, D and 3).
Figure 3
Frequencies of IL-17- and Foxp3-expressing memory subsets. Frequencies of IL-17- and Foxp3-expressing CD4+CD45RO+ (A, B) and CD4+CD45RO- T cells (C, D) in patients with atherosclerosis and controls in non-stimulated (A, C) and PMA/ionomycin-stimulated (B, D) conditions. Two of the control samples were lost after stimulation and are not included in the results
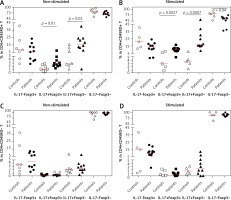
The mean fluorescent intensity (MFI) of Foxp3 and IL-17 in different T-cell subsets.
Our data showed that the MFIs of IL-17 and Foxp3 in different CD4+ T-cell subsets (CD4+CD45RO+ and CD4+CD45RO–) were not significantly different between controls and patients in non-stimulated and stimulated conditions (Figure 4). However, by considering the MFI of IL-17 in IL-17-Foxp3+, IL-17-Foxp3-, IL+17-Foxp3+, and IL-17+Foxp3- populations, we observed that only in CD4+CD45RO+ population was there a significant difference in IL-17 MFI. In this population, both before and after stimulation with PMA/ION, the MFI of IL-17 in the IL-17+Foxp3+ population was higher in patients than in controls (p = 0.04) (Figure 5).
Figure 4
The mean fluorescent intensity (MFI) of Foxp3 and IL-17 in memory and effector T-cell subsets. A – The MFIs are shown for patients and control individuals in non-stimulated and PMA/ionomycin-stimulated conditions. B – The IL-17 MFI of memory T cells based on the co-expression of IL-17 and Foxp3
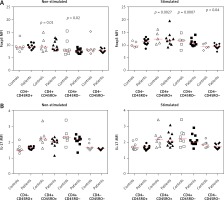
Discussion
Our results in this study confirmed that the frequency of IL-17-producing cells increases in patients with atherosclerosis in both non-stimulated and stimulated conditions. This finding is in agreement with previous studies, which showed that IL-17-producing γδ T cells [42] and Th17 increase in atherosclerosis [43]. Our results also showed that the Treg/Th17 ratio decreased in patients compared with controls. Our most interesting finding was, however, the presence of IL-17+Foxp3+CD4+ T cells in our patients, which increased in frequency after stimulation (Figure 1 B). Interestingly, this CD4+ population had a CD45RO+ (memory) but not a CD45RO- (effector) phenotype (Figure 2). Previous studies have shown an increase in circulating Th17 accompanied by a reduced number and suppression efficiency of Treg cells [16, 44]. We previously showed that the frequency of CD4+CD45RO+CD25hiFoxp3hi T-cells (activated nTregs) decreases in patients with atherosclerosis [41]. Another study showed that both resting Treg and activated Treg decrease in atherosclerosis whereas CD4+CD45RA-Foxp3lo cells, which produce IFN-γ without suppressor function, increase in patients with atherosclerosis [45]. Considering the increase in the expression of IL-17 in the CD4+CD45RO+ population and the presence of intermediate populations, it is likely that these cells are activated Tregs, which are to lose the expression of Foxp3 and become IL-17-producing CD4+CD45RO+- Foxp3- cells. This assumption is consistent with the observed higher frequency of IL-17+Foxp3+ cells in our patients.
Our findings on the presence of intermediate IL-17-producing Tregs in human atherosclerosis is new, but the presence of such populations in other inflammatory diseases is known. Indeed, since the first reports on IL-17-producing Tregs in 2009 [46], several studies have confirmed the presence of double-positive IL-17-producing Tregs, and the plasticity of Tregs and Th17 cells is now well documented [10, 47–50]. While most of these studies studied the lamina propria tissue or diseases that affect the mucosal tissues such as colon cancer, ulcerative colitis, and inflammatory bowel disease, there are other studies that have shown the conversion of Tregs to IL-17-producing cells under inflammatory conditions. A previous study showed beautifully that even Foxp3+Tregs from healthy donors can differentiate to IL-17-producing cells, and IL-17+Foxp3+ clones can be generated in vitro [10, 46]. Moreover, recent studies detected Foxp3+IL-17+ T cells in psoriatic lesions and synovia of patients with active rheumatoid arthritis [4, 10]. However, the reports on their presence in atherosclerosis are scarce.
Despite the increased frequency of CD4+IL-17+ and decreased frequency of CD4+Foxp3+ T cells in our patients, the MFI of both IL-17 and Foxp3 was lower in patients than in controls. This is consistent with the transitional phenotype and is in accordance with our previous finding that showed the mRNA expression of Foxp3 and TGF-β decrease in PBMCs of patients with atherosclerosis [41]. Moreover, it has been reported that in rheumatoid arthritis, CD25lo Tregs lose their Foxp3 expression during conversion to Th17 cells under the effect of inflammatory milieu, especially IL-6, which is a major player in the inflammatory response during atherosclerosis [10, 51].
In conclusion, our study reaffirms the increased frequency of IL-17-producing cells in atherosclerosis and suggests a reduced Treg/Th17 ratio in our patients. Our data may suggest a population shift from Foxp3+ to Foxp3+IL-17+ and Foxp3- cells in atherosclerosis; however, this assumption needs further investigation.