Introduction
Abnormal sleep pattern (ASP) is characterized by short or long durations of sleep and excessive daytime sleepiness (EDS). Obstructive sleep apnoea (OSA) is the most common form of ASP, characterized by narrowing of the upper airways (complete or partial) during sleep; EDS is one of its cardinal features [1]. During this syndrome, the change in airway muscle tone that promotes their collapse (mainly during the inspiratory phase of breathing) cannot be compensated by increasing their activity to maintain airway patency [1, 2]. OSA affects approximately one billion people all over the world and is considered as a significant and independent cardiovascular risk factor [1–3]. Unfortunately, it is often underrecognized and undertreated in everyday clinical practice [1–3]. Its actual prevalence can vary significantly according to the population that is being investigated and the definition criteria that are applied [2, 3].
Individuals with OSA experience interrupted sleep, with episodes of hypopnoea (substantial reduction of airflow, which promotes cortical arousal on electroencephalography or 3% oxygen desaturation) and/ or apnoea (full absence of airflow despite continuous respiratory activity that lasts for more than 10 s) [1, 2]. Hence, recurrent episodes of hypoxemia and hypercapnia, as well as autonomic fluctuations, are found in this population [1, 2]. Most individuals with OSA experience EDS, decreased vigilance, morning headaches, fatigue, snoring, nocturnal gasping or choking, dry mouth, drooling, nocturia, heartburn, and/or episodes of falling asleep while they are driving (leading to motor vehicle accidents) [1–3]. Diagnosis of OSA requires the following: (i) the presence of nocturnal breathing disturbances and respiratory disturbance index or apnoea-hypopnoea index (AHI) (the average amount of apnoea plus hypopnoea events/sleep hour (h)) ≥ 5; or (ii) AHI ≥ 15 events/h in the absence of clinical symptoms [1–3]. The extent of oxygen desaturations is an additional marker for evaluating the severity of this disorder [2]. AHI > 40 events/h and/or minimal oxygen saturation < 65% include the severe range of this syndrome [4].
OSA has an impact on multiple human organs and has been strongly associated with obesity, insulin resistance (IR), type 2 diabetes (T2D), non-alcoholic fatty liver disease (NAFLD)/non-alcoholic steatohepatitis (NASH), chronic kidney disease (CKD), hypertension, cerebrovascular disease, coronary artery disease (CAD), heart failure (HF), atrial fibrillation (AF), pulmonary hypertension, and all-cause mortality [2–6].
Obesity and OSA
Weight gain is an important risk factor for the development and worsening of OSA both in adults and in children; obesity also exacerbates most of its metabolic and cardiovascular comorbidities [1–3]. Approximately 40% of adults with body mass index (BMI) ≥ 30 kg/m2 suffer from this syndrome, reaching up to 98% in the morbid obese population [1, 2, 7, 8]. Abdominal obesity, rather than general obesity, is tightly associated with its development. Higher amount of visceral fat was significantly associated with OSA in non-obese individuals, who experience increased fat deposition in the neck and abdomen compared to controls [9, 10]. Indeed, waist circumference and neck size (> 43 cm in men and > 37 cm in women) have been significantly related to OSA [2, 4]. Neck size seems to be associated most with OSA even after adjusting for BMI; it has strong correlation with certain disease severity measures, such as AHI and oxygen saturation. Moreover, it has been systematically demonstrated that OSA improves significantly with weight loss [11–14].
Obesity has been associated with OSA through several mechanisms: (i) direct fat deposition within the upper airway or in its walls, which reduces the respiratory lumen; (ii) altered muscle structure, as well as fat deposition within the soft tissues, can increase upper airway collapsibility; (iii) pharyngeal shape is changed to oval, thus decreased ability for muscle contraction is observed; (iv) pharyngeal cross-sectional area is abnormally reduced and varies considerably with changes in lung volume. Suppressed tracheal traction secondary to mass effect of abdominal obesity in the chest wall was suggested as the most probable reason; (v) abdominal obesity can reduce chest compliance and functional residual capacity; and (vi) both adipocytes and macrophages secrete several adipokines and create a complex network of proinflammatory factors. These factors, as well as obesity-related leptin resistance, were shown to have somnogenic activity; they can suppress the central nervous system (CNS) and blunt upper airway neuromuscular control [1, 3, 4, 15–18].
On the other hand, mounting evidence suggests an association between chronic sleep fragmentation and cyclical episodes of hypoxia-reoxygenation with systemic inflammation, oxidative stress, sympathetic activation, hypothalamic-pituitary axis stimulation, adipokine dysregulation, and increased risk for weight gain. All these contribute to reduced insulin sensitivity, IR, compensatory hyperinsulinaemia, metabolic abnormalities, and eventually T2D, creating a vicious cycle of feed-forward effect [19–22]. Interestingly, OSA has been associated with impaired glucose metabolism in nonobese individuals (even after adjusting for generalized or visceral adiposity), while insulin-treated diabetic patients (especially women) experienced higher risk of OSA [23, 24]. Hormones related to obesity, satiety, weight control, and energy expenditure (such as leptin, adiponectin, ghrelin, and orexin) were found to be altered in individuals with OSA, while the role of gut microbiota to the pathogenesis of both obesity and OSA is under intense investigation [3, 15, 21, 22].
OSA: treatment approaches
Current therapeutic approaches mainly include body weight reduction, continuous positive airway pressure (CPAP), surgical treatment of the pharyngeal soft tissues or the facial skeleton to enlarge upper airways, and oral appliances that hold the jaw forward during sleep [25]. CPAP is undoubtedly recognized as the gold standard treatment of OSA, although treatment adherence is often suboptimal (up to 80% of individuals with OSA fail to adhere), and it does not address the pathophysiological mechanisms governing its pathogenesis, especially for overweight/obese individuals [26]. Interestingly, a large meta-analysis that explored data from 3181 patients participating in 25 randomized control trials (RCTs) suggested that CPAP promoted significant increase in body weight and BMI in this population [27].
Unfortunately, meaningful and sustained weight reduction in individuals with or at risk for T2D using lifestyle modification alone remains difficult and challenging [25, 28]. Moreover, it has been demonstrated that among obese adults with metabolic syndrome, those at high risk for OSA experience less body weight loss in response to dietary counselling, compared to adults with low risk for OSA [29]. Furthermore, individuals with OSA and higher baseline BMIs were less likely to achieve clinical remission of this disorder compared to those with lower values [30]. Hence, novel therapeutic strategies are crucially needed.
In the official clinical guidelines of the American Thoracic Society for the role of weight management in the treatment of adults with OSA it was stated that individuals with BMI ≥ 27 kg/m2 (in whom body weight has not been improved despite lifestyle modifications) can be evaluated for anti-obesity pharmacotherapy, although currently there is insufficient evidence to recommend any pharmacotherapy [31, 32]. Individuals with BMI ≥ 35 kg/m2 and OSA can be candidates for bariatric surgery [31]. A systematic review that analysed the data of 13,900 individuals participating in 69 studies showed that biliopancreatic diversion was the most effective procedure in improving or resolving OSA [33].
Material and methods
A literature search was performed systematically through Pubmed, Scope, Google, and Google Scholar (between January 2006 and October 2022), which identified relevant preclinical and clinical peer-reviewed published and ongoing studies to be included in this review. Studies in languages other than English and case reports/case series were not included. The search terms used were obesity, OSA, ASP, EDS, CPAP, glucagon-like peptide-1 (GLP-1), GLP-1 receptor (GLP-1R) agonists, liraglutide (LIRA), exenatide (EXE), semaglutide (SEMA), dulaglutide (DULA), sodium-glucose co-transporters 2 (SGLT2) inhibitors, empagliflozin (EMPA), dapagliflozin (DAPA), ertugliflozin (ERTU), canagliflozin (CANA), and luseogliflozin (LUSEO).
GLP-1 and OSA
GLP-1 and glucose-dependent insulinotropic polypeptide (GIP) are the 2 main incretins described; they are responsible for approximately 90% of the incretin effect [34]. GLP-1 is a proglucagon derived peptide. It is synthesized and secreted from the intestinal enteroendocrine L-cells (located in the distal ileum and colon) after meals in response to caloric intake. A rapid initial GLP-1 rise is found 15 to 30 min after a meal, followed by a second minor peak of its levels at 90 to 120 min [34, 35]. GLP-1 contributes to overall maintenance of glucose homeostasis through the reduction of glucagon secretion, while preclinical evidence suggests beneficial effects on pancreatic β-cell proliferation, survival, and inhibition of apoptosis [34, 36, 37].
GLP-1 promotes weight reduction through its appetite suppressant effect. This activity is mainly achieved by its direct hypothalamic activity on the arcuate nucleus (ARC). Specifically, GLP-1 has been shown to stimulate the electrical activity of the hypothalamic proopiomelanocortin neurons in the ARC by enhancing protein kinase A production and by increasing L-type calcium channel expression [38]. However, multiple areas within the CNS (such as area postrema and nucleus tractus solitarii) appear to transduce pharmacological signals that connect GLP-1R activation with suppressed food consumption and weight reduction [39]. It seems that hypothalamic response after GLP-1R activation is crucial for the effect of GLP-1R agonists on energy intake [40]. GLP-1 can also slow gastric secretion/emptying and intestinal motility, partially due to its central activity to the autonomous nervous system; it can also stimulate thermogenesis [34, 40–42]. Native GLP-1 is rapidly cleaved (half-life of 1 to 2 min) by the ubiquitously expressed serine protease dipeptidyl peptidase-4 (DPP-4) and undergoes quick renal elimination [34, 40–42].
Impairment of incretins (secretion/activity) has been described in several IR states beyond T2D, such as obesity, prediabetes, and polycystic ovary syndrome (PCOS) [34, 41, 43]. In otherwise healthy individuals and/or individuals with obesity who experience sleep disturbances, GLP-1 secretion/activity was found to be attenuated, normal, or even augmented [44–52]. In several of these studies sleep deprivation resulted in delayed postprandial GLP-1 response compared to normal sleep [48, 50, 51]. Intermittent hypoxia (mimicking sleep apnoea) was suggested to alter the composition of gut microbiota and exerted significant effects to the secretion of incretins (especially GLP-1 production) [53, 54]. However, current data are inconsistent and inconclusive, possibly because of the variety of protocols that were utilized (such as meal tolerance versus glucose tolerance, different abnormal sleep patterns, and timing of GLP-sampling) and the heterogenous populations enrolled in the above studies [44–52].
The association between OSA and incretin secretion/activity was investigated in 2 main studies [55, 56]. In the first study, 96 patients without diabetes were enrolled of whom (i) 21 patients had mild or non-OSA; (ii) 38 experienced moderate OSA; and (iii) 37 had severe OSA [55]. Waist circumference, BMI, and fasting insulin levels were significantly higher in patients with severe OSA compared with those in the other 2 arms. AHI was found to be an independent determining factor of fasting GLP-1 levels, while DPP-4 activity was not associated with OSA parameters and severity. In patients with very severe OSA (AHI > 50 events/h), postprandial GLP-1 levels were decreased, but fasting GLP-1 levels were significantly increased (although the association between OSA and GLP-1 response was lost after adjusting for waist circumference). In the other study, 71 individuals with abnormal glucose tolerance were enrolled (including individuals with prediabetes and 15 patients with early T2D) [56]. OSA evaluation was available in 69 participants, of whom 71.4% were finally diagnosed (59.1% experienced mild, 34.6% moderate, and 6.1% severe OSA). No associations were shown among fasting GLP-1 levels and the area under the curve (AUC) for GLP-1 with sleep duration, sleep efficiency, or their variability. However, higher OSA severity was associated with lower GLP-1 response to glucose, after adjusting for BMI, sex, and glycaemic condition (p = 0.045). It was suggested that reduced GLP-1 response in more severe forms of OSA could further deteriorate abnormal glucose metabolism, cause weight gain, and increase the risk of developing T2D.
GLP-1R agonists
GLP-1R agonists exert GLP-1 activity and are resistant to DPP-4 degradation; they have achieved significantly lower glycated hemoglobulin (A1C) levels in patients with T2D and promoted remarkable weight loss [57]. Abdominal fat loss, as well as sustained reductions of fasting insulin levels and markers of IR, have also been shown in patients with T2D and individuals with overweight/obesity and/or prediabetes after their administration [58–60]. Additionally, GLP-1R agonists have reduced all-cause and cardiovascular mortality in patients with T2D and high cardiovascular risk, as well as urinary albumin excretion; they predominantly affected macroalbuminuria, whereas their possible effects on hard renal endpoints are less clear [61, 62].
LIRA and SEMA gained approval from the Food and Drug Administration and the European Medicines Agency for weight loss in obese individuals or people who are overweight with BMI ≥ 27 kg/m2 and have experienced at least one weight-associated comorbid condition (such as prediabetes, hypertension, dyslipidaemia, and OSA) combined with lifestyle modifications [63]. SEMA was recently suggested to be the most effective of its category in terms of weight loss; it can penetrate certain CNS regions, which are essential for appetite control and hunger and are not protected by the blood-brain barrier. In this way it can directly and indirectly alter the activity of neural pathways involved in food intake and energy expenditure and stimulate multiple cellular pathways engaged in providing input to key appetite-modulating relay areas [64, 65]. Furthermore, the escalation of DULA given in a weekly dose of 3 mg or 4.5 mg has achieved further incremental reductions of body weight, regardless of baseline A1c or BMI, compared with once-weekly 1.5 mg in patients with T2D [66]. These beneficial effects were suggested to be particularly helpful in other IR-associated disorders beyond T2D, such as individuals with NAFLD/NASH and PCOS [65, 67, 68]. Hence, their possible role in individuals with OSA has become a field of intense research.
Liraglutide
A retrospective observational study investigated 158 obese patients with T2D who were treated with LIRA (starting from a daily dose of 0.6 mg that was increased over 1 week to 1.2 mg/day) at least 3 months before study enrolment [69]. The diagnosis of OSA was not deemed necessary for inclusion in the study. None of the patients enrolled were treated with CPAP during the study period. The Epworth Sleepiness Scale (ESS) score was applied in order to estimate the clinical impact on EDS. ESS score data were retrospectively analysed from the medical records at 3 time points: at LIRA initiation (baseline) and at months one and 3 after LIRA initiation. After 3 months of LIRA therapy, significant reductions of body weight (102.8 ±17.9 kg vs. 98.4 ±16.9 kg, p < 0.001), BMI (39.6 ±6.8 kg/m2 vs. 37.9 ±6.4 kg/m2, p < 0.001), waist circumference (122.1 ±14.0 cm vs. 118.9 ±13.3 cm, p < 0.001), and neck circumference (42.2 ±3.3 cm vs. 40.8 ±3.3 cm, p < 0.005) were described. ESS score was significantly reduced from baseline to month 1 (p < 0.001) and was maintained after 3 months (p < 0.001). Body weight loss was the only variable related to changes in ESS score (p < 0.05) and not total body fat mass. This was the first published study to demonstrate a significant association between body weight loss after LIRA therapy and EDS improvement in obese patients with T2D. The main limitations if this study were as follows: (i) the retrospective origin; (ii) data from sleep studies were not available; and (iii) the lack of a comparator arm.
The SCALE Sleep Apnoea RCT was a 32-week multicentre study, which investigated whether LIRA in a daily dose of 3 mg could suppress the severity of OSA (as an adjunct to lifestyle modifications) in 359 non-diabetic obese individuals with moderate (AHI 15–29.9 events/h) or severe (AHI ≥ 30 events/h-approximately 67% of the participants) OSA, who were unwilling or unable to use CPAP therapy [70]. LIRA was started from a daily dose of 0.6 mg and was escalated in weekly 0.6-mg increments to the maximum dose of 3.0 mg at the fourth week; the maximum dose was maintained for another 28 weeks. Eventually, 276 participants (LIRA: 134 (74%) vs. placebo: 142 (79%)) completed the study. After 32 weeks, mean weight reduction was significantly higher during LIRA therapy versus placebo (–5.7 ±0.4% vs. –1.6 ±0.3%, p < 0.001). Significantly higher reductions in waist and neck circumference, as well as BMI, were reported after LIRA therapy compared with placebo. The mean reduction of AHI after LIRA administration was significantly higher than placebo and was approximately 6 events/h (–12.2 ±1.8 events/h vs. –6.1 ±2.0 events/h, p = 0.015). Greater improvement of AHI was found with greater weight loss; this effect was more pronounced in individuals with severe OSA at baseline. There was also a trend for more LIRA-treated than placebo-treated individuals who did not longer meet the diagnostic criteria for OSA (p = 0.07) or experienced 50% reduction of their baseline AHI score (31.5% vs. 21.7%, respectively, p = 0.05). Greater weight reduction at the end of the study was also significantly related to higher improvements in end points associated with sleep architecture, oxygen saturation, total scores on ESS, and self-reported quality of life (Functional Outcomes of Sleep Questionnaire (FOSQ)). Weight reduction after LIRA administration did not plateau. Hence, it is possible that further weight reduction-associated improvement of AHI could have been found with longer LIRA therapy. More individuals reported side effects after LIRA therapy (80.1%) than after placebo (69.3%), driven by the higher prevalence of gastrointestinal adverse effects, as expected (mainly nausea). Gastrointestinal adverse effects were generally transient and mild or moderate in severity; they were generally described within the initial 8 weeks of therapy. Mean resting heart rate (HR) was increased by 2 beats/minute (min) after LIRA administration compared to placebo. Since individuals with OSA experience increased sympathetic activity, it is reassuring that the increase in HR after LIRA was similar or even smaller compared to HR values previously described with LIRA (for the same doses) in individuals without OSA [71].
Three studies have been launched that are exploring the possible role of LIRA in individuals with OSA:
The ROMANCE study is an outpatient, single-centred, randomized, open-labelled, phase IV study, in which 132 obese patients with T2D and newly diagnosed OSA (AHI ≥ 15 events/h) will be enrolled [72]. Patients will be divided in 4 treatment arms for 26 weeks: (i) LIRA in a daily dose of 1.8 mg; (ii) LIRA in a daily dose of 1.8 mg together with CPAP; (iii) CPAP alone (conventional care); or (iv) no treatment (control). The aim of the trial is for 128 patients to complete it (n = 32 per study group). The primary objective is to determine whether LIRA administration can be beneficial in this population, either as monotherapy or combined with CPAP. The primary outcome is the change of AHI from baseline values. Major secondary objectives are the change of A1C from baseline and the change of total body weight. Changes in daytime sleepiness using the ESS score, quality of life, and assessment of treatment compliance will be also explored.
The activity and financial burden of practicing the administration of LIRA in a daily dose of 3.0 mg given to individuals with obesity (STRIVE study, NCT03036800) is a 2-year, real-world, open-label, randomized control trial investigating the administration of LIRA in a daily dose of 3 mg on top of standard of care (provided in specialist weight-management services) in 384 individuals having BMI ≥ 35 kg/m2 plus either prediabetes, hypertension, T2D, or OSA; efficacy targets and prespecified stopping rules, when these targets are not achieved, will be evaluated [73]. The primary objective will be to compare the proportion of individuals achieving weight loss ≥ 15% at 52 weeks between the 2 arms. The secondary objectives are to compare the effects on obesity-related complications (prediabetes, T2D, hypertension, depression, OSA, dyslipidaemia, depression) at 52 and 104 weeks.
An explorative, proof-of-concept study is active and is investigating the possible role of LIRA (starting at a daily dose of 0.6 mg with weekly 0.6-mg increments up to 3 mg combined with lifestyle modification) in non-diabetic individuals with moderate to severe OSA compared with standard CPAP or the combination of both on AHI, blood pressure (BP), metabolic parameters, endothelial function, coronary artery calcification, and vascular inflammation (NCT04186494) [74].
Exenatide
A recent study investigated the possible effects of EXE (0.5 μg/kg as a single dose for 9 days) in 40 male, rapid eye movement (REM) sleep-deprived Wistar rats; data for memory performance, anxiety/depression like behaviour, markers of oxidative stress, and synaptic protein levels were evaluated [75]. Memory performance was significantly reduced after REM sleep deprivation for 24 h. EXE administration improved memory performance for 24 and 48 h after REM sleep deprivation. Furthermore, longer REM sleep deprivation for 72 h significantly stimulated excitability, depression-like behaviour, and exploratory behaviour. EXE administration normalized bot excitability and exploratory behaviour but failed to improve depression-like behaviour. Increased levels of hippocampus calcium/calmodulin dependent kinase II (CaMKII-a major regulator of hippocampal learning, memory, synaptic plasticity, and long-term potentiation) were suggested as the main mechanism though which EXE exerted its beneficial effects [76]. Both REM sleep deprivation and EXE therapy had no effect on the oxidant/antioxidant status in the hippocampus and in several other brain areas.
An exploratory, placebo-controlled, 22-week study investigated the possible role of EXE administration to 8 obese patients with T2D (mean age: 50 ±4.9 years) on EDS, driving performance, and depression score [77]. All patients enrolled were evaluated at baseline, one week after a 10-week saline (placebo) administration, and one week after 10-weeks of EXE therapy. EXE was started at 5 μg twice daily and titrated to 10 μg twice daily within 4 weeks. The OSLER test was applied for the estimation of objective sleepiness and sustained attention. Driving performance was evaluated by a driving simulator. ESS score, assessment of depressive mood (Beck Depression Inventory scale), and quality-of-life questionnaire were also used. There was a trend towards reduction of body weight, A1c, and BMI after EXE administration, which did not reach statistical significance. EXE reduced both subjective sleepiness (based on the ESS score evaluation) and objective sleepiness. Specifically, sleep latency was significantly increased between the placebo and EXE arms after adjusting for changes in body weight, A1C, and depression scores. Adjusted mean sleep latency times were 32.1 ±1.7, 29.1 ±1.7, and 37.7 ±1.7 min for baseline, placebo, and EXE, respectively (p = 0.002). Significantly increased values between baseline and EXE arms (p = 0.021) and between placebo and EXE arms (p = 0.001) were shown. Improvement of mean sleep latency time could be predicted by changes in body weight (p = 0.003), but not A1C changes. No significant changes were reported in driving performance and cytokine reduction. Furthermore, significant reductions in depression scores between baseline and EXE groups were shown; non-significant reductions in depression scores were also estimated between placebo and EXE arms. Because body weight reduction could only partially explain these findings, it was suggested that EXE could improve EDS through its direct activity on the hypothalamus (justifying also the trend towards reduction in depression scores). The main limitations of this study were as follows: (i) the small size; (ii) the short duration; and (iii) the lack of sleep data at baseline and during different phases of the study. Major results of the main clinical studies (published and ongoing) in which GLP-1R agonists were administered in individuals with ASP are shown in Table I.
Table I
GLP-1R agonists and OSA: major results of main clinical studies (published and ongoing), in which they were administered to individuals with ASP
Ref. | Year* | Study population | Main results/objectives |
---|---|---|---|
Idris et al. [77] | 2013 | An exploratory, placebo-controlled, 22-week study explored the possible role of EXE administration to 8 obese patients with T2D and EDS without OSA. All patients enrolled were evaluated at baseline, 1 week after a 10-week saline (placebo) administration, and one week after a 10-week EXE therapy. EXE was started at 5 μg twice daily and titrated to 10 μg twice daily within 4 weeks of therapy | EXE reduced both subjective sleepiness (based on the ESS evaluation) and objective sleepiness as estimated by the OSLER test. Mean sleep latency time improvement could be predicted only by changes in BW (p = 0.003). Significant reductions in depression scores between baseline and EXE groups were shown; non-significant reductions in depression scores were estimated between placebo and EXE arms |
Gomez-Peralta et al. [69] | 2015 | A retrospective observational study investigated 158 obese patients with T2D and EDS, who were treated with LIRA (maximum 1.2 mg/day), at least 3 months before enrolment. OSA was not necessary for inclusion. ESS score was applied to estimate the clinical impact to EDS | ESS score was significantly reduced from baseline to month 1 (p < 0.001) and was maintained after 3 months of LIRA therapy (p < 0.001). However, the only variable, which was related to changes in ESS score, was BW loss (p < 0.05), not total body fat mass |
Blackman et al. [70] | 2016 | The SCALE Sleep Apnoea RCT was a 32-week multicentre RCT, which investigated whether LIRA in a daily dose of 3 mg could suppress the severity of OSA in 359 non-diabetic obese individuals with moderate or severe OSA unwilling or unable to use CPAP therapy. LIRA was titrated in the maximum dose of 3.0 mg at the fourth week | Mean reduction in AHI after LIRA administration was significantly higher versus placebo and was approximately six events/h (–12.2 ±1.8 events/h versus –6.1 ±2.0 events/h, p = 0.015). Greater improvements in AHI were found with greater BW loss; this effect was more pronounced in individuals with severe OSA at baseline |
Sprung et al. [72] | 2020 | The ROMANCE study is an outpatient, single-centred, randomized, open-labelled, phase IV study, in which 132 obese patients with T2D and newly diagnosed OSA are planned to be enrolled. Patients will be divided in 4 treatment arms for 26 weeks: (i) LIRA in a daily dose of 1.8 mg; (ii) LIRA in a daily dose of 1.8 mg together with CPAP; (iii) CPAP alone (conventional care); or (iv) no treatment (control) | The aim of the trial is completion by 128 patients (n = 32 per study group). The primary objective is to determine whether LIRA administration can be beneficial in this population, either as monotherapy or combined with CPAP. The primary outcome measure is the change in AHI from baseline values |
Papamargaritis et al. [73] | 2020 | The activity and financial burden of practicing the administration of LIRA in a daily dose of 3.0 mg, to individuals with obesity (STRIVE study, NCT03036800) is a 2-year, real-world, open-label RCT investigating the administration of LIRA (in a daily dose of 3 mg) on top of standard of care in 384 individuals having BMI ≥ 35 kg/m2 plus either prediabetes, hypertension, T2D, or OSA | One of the secondary objectives is to compare the effects of LIRA administration in this population at 52 and 104 weeks |
Clinical Trials.gov al. [74] | 2022 | An explorative, proof-of-concept study (NCT04186494) is active and is investigating the possible role of LIRA (up to 3 mg combined with lifestyle modification) in non-diabetic patients with moderate to severe OSA versus standard CPAP or the combination of both | AHI, BP, metabolic parameters, endothelial function, coronary artery calcification, and vascular inflammation will be evaluated |
LIRA – liraglutide, EXE – exenatide, T2D – type 2 diabetes, OSA – obstructive sleep apnoea, EDS – excessive daytime sleepiness, ASP – abnormal sleep pattern, ESS – Epworth Sleepiness Scale, CPAP – continuous positive airway pressure, AHI – apnoea-hypopnoea index, BW – body weight, BP – blood pressure, RCT – randomized control trial, BMI – body mass index, Ref. – reference,
Sodium-glucose co-transporter inhibitors
Introduction
SGLT2 are found in the early segment one (S1) to the brush-border membrane of the proximal convoluted tubule (PCT) and are responsible for about 90% of filtered glucose reabsorption – the remaining amount in the glomerular filtrate is salvaged by SGLT1 in the S3 of the PCT [78]. The upregulation (activity and number) of SGLT2 in patients with T2D contributes to approximately 20% to 30% higher tubular maximum renal glucose reabsorptive capacity (TmG) and enhanced renal threshold for glucose excretion (RTG). This in turn creates a vicious cycle and contributes to the pathogenesis and maintenance of high glucose levels [78–81].
SGLT2 inhibitors promote approximately 50% reduction in TmG and cause significant glycosuria [78, 79]. They have achieved median A1C reductions of 0.6–0.8% in patients with T2D and normal renal function, while a more pronounced effect was found in patients who experienced baseline A1c levels ≥ 10% [78, 80]. SGLT2 inhibitors have achieved major cardiovascular and renal benefits in patients with T2D and drastically changed the landscape of their treatment. They signified a new era, in which treatment strategies should be tailored based on end-organ protection and patient comorbidities, rather than focusing only on reducing blood glucose levels [78, 82]. Their cardiovascular and renal benefits have been also established in the non-diabetic population with CKD and/or HF [83].
Both preclinical and clinical studies of SGLT2 inhibitors have consistently demonstrated significant body weight reduction (significant loss of 240–320 calories/day resulting in an average weight loss of 2–4 kg during the first 24 weeks). Systolic BP levels were also decreased after their administration [84]. Through increased fatty acids oxidation and ketogenesis, SGLT2 inhibitors reduced adipose tissue and liver steatosis; enhanced lipolysis and visceral fat loss (reaching up to 70% of total weight reduction) have been reported [85, 86]. Moreover, several studies have suggested that this drug class possess significant anti-inflammatory and anti-oxidative stress properties [78, 80]. Hence, their possible role in other metabolically related disorders is currently under intense investigation. Encouraging preclinical and clinical evidence is also growing that suggests beneficial effects of SGLT2 inhibitors to other IR-related disorders beyond a diabetic state (such as obesity, prediabetes, PCOS, and NAFLD/NASH) [87]. The beneficial effects of this drug class on metabolic outcomes (body weight, BP control) were also reported in a recent meta-analysis of RCTs in non-diabetic individuals [88].
Data from meta-analyses
Two recent meta-analyses, which explored any possible adverse events after therapy with SGLT2 inhibitors, have shown that this drug class was associated with reduced risk of developing OSA [89, 90]. In the first meta-analysis, which analysed data from 9 major studies (33,124 individuals in the SGLT2 inhibitor arm vs. 26,568 individuals in the placebo arm) found that the relative risk (RR) for experiencing OSA after their administration was 0.36 (p = 0.023) [89]. The second meta-analysis investigated data from 9 cardiorenal outcome studies that were stratified by 2 different doses of SGLT2 inhibitors (high or low dose); all of them had large sample sizes (ranging from 3730 to 17,160 patients). It was suggested that SGLT2 inhibitors administered either at low dose or high dose were associated with RR of 0.37 for developing OSA (95% CI: 0.17–0.81; I 2 = 0) [90]. Another meta-analysis, in which data from 9 large RCTs were analysed to evaluate the possible associations of several respiratory disorders and SGLT2 administration, suggested that SGLT2 inhibitors reduced the occurrence of OSA by 65% compared with placebo (RR = 0.35; I 2 = 0, p for treatment effect: 0.073) [91]. Neither the presence of T2D nor CKD affected the activity of SGLT2 inhibitors on the evolution of OSA syndrome. Furthermore, a small meta-analysis explored data form 65 T2D patients with OSA participating in 3 studies (2 prospective and one retrospective) [92]. DAPA was the main SGLT2 inhibitor administered (73.8%). The mean duration of treatment was of 24.6 ±3 weeks. It was suggested that SGLT2 inhibitors can decrease AHI, BMI, and systolic BP, while oxygen saturation (SpO2) was increased. However, these data were not statistically significant. High heterogeneity of several variables was one of the main limitations of this study.
Pathophysiological concepts
The possible mechanisms that were currently described in order to explain the possible beneficial effects of SGLT2 inhibitors in individuals with OSA were as follows: (i) SGLT2 inhibitors promote body weight reduction and especially visceral fat. Reduction of fat deposits around the thorax and neck can also improve airway collapsibility; (ii) due to osmotic diuresis, natriuresis, and preservation of renal function, they can reduce systemic fluid retention and hypervolaemia. In this way, SGLT2 inhibitors may decrease rostral-to-caudal fluid shifts in the recumbent sleep position, cardiac preload, and intrathoracic fluid retention; (iii) due to the modest increase in urinary frequency, manifested usually as nocturia, SGLT2 inhibitors could promote interrupted sleep cycles and suppress the duration of REM sleep (a higher risk period for OSA events); (iv) SGLT2 inhibitors can suppress circadian sympathetic nervous system activation and its related conditions (such as non-dipping pattern of hypertension, cardiac autonomic neuropathy, and stroke); (v) forced glucosuria after their administration can reduce the availability of glucose as an energy fuel. In this way other substrates are utilized, and less endogenous CO2 production is expected, with less CO2 to be removed by the respiratory tract; and (vi) modulation of arousal threshold, muscle compensation, and loop gain [78, 93–98].
Empagliflozin
BI 10773 (Empagliflozin) Cardiovascular Outcome Event Trial in Type 2 Diabetes Mellitus Patients (EMPA-REG OUTCOME) was a pivotal phase III RCT, in which 7020 patients with T2D at high cardiovascular risk and estimated glomerular filtration rate (eGFR) ≥ 30 ml/min/1.73 m2 were randomized to receive either EMPA (10 or 25 mg daily, n = 2333) or placebo (n = 4687), against a background of standard glucose care [99]. A rapid reduction (less than 3 months) of the primary composite outcome (death from cardiovascular causes, nonfatal myocardial infarction (MI) or nonfatal stroke) by 14% (p = 0.04) was reported after EMPA administration, with an impressive 38% reduction of the relative risk of death from cardiovascular causes compared to placebo (p < 0.001). It also achieved 39% reduction of the main renal outcome (progression to macroalbuminuria, doubling of serum creatinine level combined with eGFR ≤ 45 ml/min/1.73 m2, initiation of renal replacement therapy or renal associated death) and 35% reduction in hospitalization for HF (p = 0.002).
A recent exploratory post-hoc analysis of the EMPA-REG OUTCOME trial demonstrated that about 6% of the population enrolled experienced OSA at baseline [100]. Patients with OSA were more likely to experience moderate to severe obesity (55.2% vs. 18.2%), had greater prevalence of CAD, longer duration of T2D, higher rates of insulin administration, and more prevalent use of antihypertensive therapies. They had an increased risk of developing cardiovascular and kidney events (as well as higher overall all-cause mortality) compared to those without OSA, confirming the huge cardiorenal burden that characterizes this population. A trend towards enhanced EMPA activity on weight loss (adjusted for baseline body weight) in patients with OSA compared to those without OSA was also reported. There was also a signal for higher magnitude of benefit among patients with OSA at baseline compared to those with no OSA, as far as the reduction of the progression to macroalbuminuria was concerned (p = 0.03). Moreover, patients treated with EMPA were 52% less likely to develop new OSA than those treated with placebo, although fewer patients with BMI > 35 kg/m2 were included in the EMPA arm than placebo (n = 143 vs. n = 73). Interestingly, when mediation analysis was performed to investigate further what factors may have contributed to the reduced risk of developing new OSA, only 22% of the lower risk was attributable to combined changes in conventional risk factors (with the majority being due to weight reduction). It should be stated that OSA diagnosis was based on patient/investigator report, rather than objective assessment using systematic prospective polysomnography, and thus AHI measurements were lacking. Additively, more patients with OSA in the EMPA arm received either diuretics (n = 170) or metformin (MET, n = 188) than those in the placebo arm (n = 74 and n = 81, respectively), which could affect body weight differences.
Ertugliflozin
The Evaluation of Ertugliflozin Efficacy and Safety Cardiovascular Outcomes Trial (VERTIS CV) was a multicentre RCT in which 8246 patients with T2D and atherosclerotic cardiovascular disease were randomized to receive either 5 mg or 15 mg of ERTU versus placebo once a day; ERTU was reported to be noninferior to placebo with respect to the composite outcome of cardiovascular death, nonfatal MI, or nonfatal stroke [101]. An exploratory analysis showed that approximately 7% of the population enrolled experienced baseline OSA. Among patients without baseline OSA, there was a 48% reduction for incident OSA in those treated with ERTU versus placebo (p = 0.04) [102]. There was an early differentiation in the incidence of OSA (from the first 12 months of the study) between patients treated with ERTU versus placebo. A recent meta-analysis, which analysed data from the EMPA-REG OUTCOME trial and the VERTS CV study, suggested that the administration of empagliflozin/ertugliflozin significantly reduced the risk of developing new-onset OSA by 47% (p = 0.003) [103].
Dapagliflozin
Two small prospective studies explored the possible role of DAPA in individuals with sleep disordered breathing (SDB) [104, 105]. In the first study DAPA (in a daily dose of 5 mg) was administered in 30 obese patients with T2D for 24 weeks [104]. SDB was defined by using 3% oxygen desaturation index (ODI). Individuals who experience 5–14 events/h were considered to have mild SDB (80% of the population enrolled), while those with at least 15 events/h were defined as moderate to severe SDB. After 24 weeks of therapy weight loss was 1.7 kg and 2.56 kg among patients with mild and moderate/severe SDB, respectively. DAPA therapy achieved significant improvements in 3% ODI only in the group of moderate/severe SDP (25.0 ±3.8 at baseline versus 18.5 ±6.1 at the end of the study, p = 0.017). Interestingly, the sensitivity analysis that was performed suggested that neither body weight loss nor neck circumference reduction were related to the improvement in 3% ODI. The lack of polysomnography and data for AHI, as well as the absence of a control group, were the main limitations of this study.
In the second study 36 patients with OSA and T2D were divided into 2 arms: (i) DAPA arm (n = 18) – all patients received DAPA in a daily dose of 5 mg, which was increased to 10 mg 1 week later; and (ii) control arm (n = 18) – all patients received glimepiride (GLIME) in a daily dose of 2 mg, which could be titrated up to 4 mg if the glycaemic control was not satisfactory [105]. Both groups received MET 850 mg twice a day. Total treatment duration was 24 weeks. DAPA achieved significant reductions in BMI and Homeostatic Model Assessment for IR (HOMA-IR) compared to GLIME (p < 0.05). It also achieved significant reductions in AHI, higher minimum SpO2, and decreased ESS scores than the control arm (p < 0.05). Because MET has not achieved improvements of ventilatory function in T2D patients with OSA, it was suggested that DAPA played major role in this finding [106, 107]. The main limitations of this study were the small number, the short duration, and the absence of data about the effect of DAPA on neck circumference and other obesity-related signs. Moreover, the use of sulfonylurea in the control group potentially influenced the reported difference in BMI values.
Several SGLT-2 inhibitors
The possible activity of SGLT2 inhibitors (n = 9) compared to other hypoglycaemic medications (n = 7) in patients with T2D and OSA, who initiated CPAP therapy during their administration, was investigated in a recent 3-month study [108]. Participants in the SGLT2 arm received EMPA 10 mg/day, LUSEO 2.5 mg/day, and DAPA 5 mg/day. Significantly higher BMI and body weight were found in the SGLT2 arm compared to baseline values. However, SGLT2 inhibitors had been administered continuously for 6 months before the initiation of CPAP. Hence, weight gain was suggested to be the result of OSA improvement following the initiation of CPAP therapy. No significant changes of these parameters were found in the non-SGLT2 inhibitors group. No significant changes of A1c levels were described in both arms. AHI was significantly reduced 3 months after CPAP therapy in both groups, with no significant difference between them.
A small retrospective study (without a control group) investigated the possible effects of SGLT2 inhibitors in 18 T2D patients with OSA regarding weight reduction and changes in AHI [109]. SGLT2 inhibitors were administered for a median of 21 weeks, and 12 patients were diagnosed with severe OSA. Body weight and BMI were significantly reduced after therapy with SGLT2 inhibitors. AHI was reduced from 31.9 ±18.0 to 18.8 ±11.5 events/h after their administration (p = 0.003). The number of participants with severe OSA was reduced from 12 to 4. However, correlation analysis suggested that more body weight reduction was associated with less AHI improvement in patients with severe OSA. Compensatory hyperphagia and concurrent diuretic therapy were some of the main explanations that were suggested in order to justify this finding. The main results of clinical studies, in which SGLT2 inhibitors were administered in individuals with ASP, are shown in Table II.
Table II
SGLT2 inhibitors and OSA: main results of current clinical studies, in which they were administered to individuals with ASP
Ref. | Year* | MED | Study population | Main results |
---|---|---|---|---|
Furukawa et al. [104] | 2018 | DAPA | DAPA in a daily dose of 5 mg was administered in 30 obese patients with T2D and SDB for 24 weeks. SDB was defined by 3% ODI level. Individuals who experience 5–14 events/h were considered to have mild SDB, while those with at least 15 events/h were defined as moderate to severe | DAPA therapy achieved significant improvement in 3% ODI only in the group with moderate/severe disorder (p = 0.017). Interestingly, the sensitivity analysis that was performed suggested that neither BW loss nor neck circumference reduction were related to 3% ODI improvement |
Sawada et al. [109] | 2018 | SGLT2 inhibitors | A small retrospective study investigated the possible effects of SGLT2 inhibitors in BW loss and change in AHI in 18 T2D patients with OSA (median duration of therapy was 21 weeks) | AHI was reduced from 31.9 ±18.0 to 18.8 ±11.5 events/h after the administration of SGLT-2 inhibitors (p = 0.003). The number of participants with severe OSA was reduced from 12 to 4 |
Tang et al. [105] | 2019 | DAPA | 36 patients with T2D and OSA were divided into 2 arms: (i) DAPA arm (n = 18) – all patients received DAPA in a daily dose of 5 mg, which was increased to 10 mg 1 week later; and (ii) control arm (n = 18) – all patients received GLIME in a daily dose of 2 mg, which could be titrated up to 4 mg if the glycaemic control was not satisfactory. Both groups received MET in a daily dose of 850 mg twice a day. The total treatment duration was 24 weeks | DAPA achieved significant reductions in BMI and HOMA-IR compared to GLIME (p < 0.05). It also achieved significant reduction in AHI, increase in LSpO2 levels, and decrease in ESS score versus the control arm (p < 0.05) |
Neeland et al. [100] | 2020 | EMPA | Exploratory post-hoc analysis of the EMPA-REG OUTCOME trial | 6% of the population enrolled had OSA at baseline. A trend towards higher EMPA activity on BW loss, in patients with OSA versus those without OSA, was reported. There was also a signal for higher magnitude of benefit among those patients with OSA at baseline versus no OSA, for the reduction of the progression to macroalbuminuria (p = 0.03). Moreover, patients treated with EMPA were 52% less likely to develop new OSA versus those treated with placebo. Interestingly, only 22% of the lower risk was attributable to combined changes in conventional risk factors (with the majority being due to BW reduction) |
Kusunoki et al. [108] | 2021 | EMPA, LUSEO, DAPA | The possible activity of SGLT2 inhibitors (n = 9) compared to other hypoglycaemic medications (n = 7) in patients with T2D and OSA, who initiated CPAP therapy during their administration, was investigated in a 3-month study | AHI was significantly reduced after 3 months of CPAP therapy in both groups, with no significant difference between the 2 groups |
Wojeck et al. [102] | 2022 | ERTU | Exploratory post-hoc analysis of the VERTIS CV trial | 7% of the population enrolled experienced baseline OSA. Among the patients without baseline OSA, there was 48% RR reduction for incident OSA after ERTU therapy versus placebo (p = 0.04). There was an early differentiation in the incidence of OSA, between patients treated with ERTU versus placebo, beginning from the first 12 months of the study |
EMPA-REG OUTCOME trial: BI 10773 (Empagliflozin) Cardiovascular Outcome Event Trial in Type 2 Diabetes Mellitus Patients; OSA – obstructive sleep apnoea, AHI – apnoea-hypopnea index, CPAP – continuous positive airway pressure, SGLT2 – sodium-glucose co-transporters 2, EMPA – empagliflozin, DAPA – dapagliflozin, ERTU – ertugliflozin, LUSEO – luseogliflozin, GLIME – glimepiride, MET – metformin, SDB – sleep disordered breathing, ODI – oxygen desaturation index, BW – body weight, RR – relative risk, LSpO2 – minimum oxygen saturation, ESS – Epworth Somnolence Scale, HOMA-IR – Homeostatic Model Assessment for Insulin Resistance, MED – medication, Ref. – reference,
Limitations
The limitations of this review are the limitations that exist in current literature. These are mainly as follows: (i) the short period of research; (ii) the small number of individuals investigated; (iii) the absence of a control arm (in some of these studies); (iv) the retrospective design of some studies; (v) the lack of polysomnography and data for AHI in several studies; (vi) the absence of data about neck circumference and other obesity-related signs; and (vii) differences in some variables between the 2 groups that can affect the final results of the studies [such as BMI and certain medications (diuretics, MET).
Conclusions
OSA is one of the most common and serious sleep-related breathing disorders, which affects cardiovascular morbidity and mortality, as well as the quality of life [1, 3, 110]. It is associated with substantial economic costs both for the individuals and society as a whole [110]. The pandemic of obesity is sweeping across the world and is a fundamental risk factor for its evolution. Pharmacological treatments that differentially target the pathophysiological mechanisms governing both obesity and OSA have been tested with some success, either as monotherapy or as adjunct therapies to other therapeutic approaches; GLP-1R agonists and SGLT-2 inhibitors are promising tools in our therapeutic armamentarium in this setting [111–113] (Figure 1).
Figure 1
GLP-1RA, SGLT2i and OSA: Possible pathophysiological mechanisms of protection. 1 – OB is an important risk factor for the development and worsening of OSA; it also exacerbates most of its metabolic and cardiovascular comorbidities. Reduction of the respiratory lumen, increased upper airway collapsibility, suppressed tracheal traction secondary to mass effect of abdominal OB, and decreased chest compliance and functional residual capacity are several potential mechanisms. 2 – Chronic sleep fragmentation and cyclical episodes of hypoxia-reoxygenation during OSA promote systemic inflammation, oxidative stress, sympathetic activation, hypothalamic-pituitary axis stimulation, adipokine dysregulation and increased risk for weight gain. These in turn contribute to IR, compensatory hyperinsulinemia, metabolic abnormalities and eventually T2D, creating a vicious cycle of feed-forward effect. 3 – GLP-1RA have achieved remarkable reductions of body weight in patients with T2D, individuals with overweight/OB and/or prediabetes. Body weight loss was the only significant factor associated with improvement of AHI and other sleep-related scores in individuals with OSA. 4 – The well-recognized pleotropic effects of GLP-1RA (normalization of neurogenesis and synaptic plasticity, protection against neuronal apoptosis, and suppression of increased oxidative stress), in conjunction with the presence of GLP-1Rs in the hypothalamic nuclei that regulate sleep and wakefulness, suggest several possible direct effects of GLP-1RA in affecting sleepiness and other related parameters (memory, anxiety, and depression). 5 – SGLT2i have consistently demonstrated significant body weight reduction (average weight loss of 2–4 kg during the first 24 weeks). Through increased fatty acids oxidation and ketogenesis SGLT2i reduced adipose tissue and liver steatosis; enhanced lipolysis and visceral fat loss (reaching up to 70% of total weight reduction) have been reported. 6 – Reduction of fat deposits around the thorax and neck, reduction of rostral-to-caudal fluid shifts in the recumbent sleep position, suppression of circadian sympathetic nervous system activation, and suppressed availability of glucose as an energy fuel are some possible pathophysiological mechanisms described in order to justify their possible beneficial effects to individuals with OSA. GLP-1RA – glucagon-like peptide-1 agonists, SGLT2i – sodium-glucose co-transporters 2 inhibitors, OSA – obstructive sleep apnoea, IR – insulin resistance, T2D – type 2 diabetes, AHI – apnoea-hypopnoea index, OB – obesity
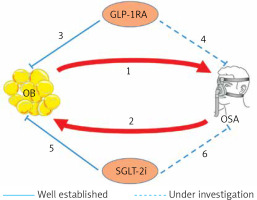
GLP-1R agonists have demonstrated interesting results in individuals with ASP (with or without OSA), while results from future RCTs and post hoc analyses from other published RCTs are anticipated (Table I). Body weight loss was the only significant factor associated with improvement of AHI and other sleep-related scores in current literature. However, the well-recognized pleotropic effects of GLP-1R agonists (normalization of neurogenesis and synaptic plasticity, protection against neuronal apoptosis, and suppression of increased oxidative stress), in conjunction with the presence of GLP-1Rs in the hypothalamic nuclei that regulate sleep and wakefulness, suggest several possible direct effects of GLP-1R agonists in affecting sleepiness and other related parameters (memory, anxiety, and depression) [77, 114]. Furthermore, GLP-1R agonists could be administered as adjunct therapy to weight reduction in individuals with prior bariatric surgery, although a recent study suggested that LIRA did not promote the resolution of other obesity-related comorbidities (including OSA) compared to placebo in this population [115, 116]. However, this study included a small sample size (LIRA: n = 12 vs. placebo: n = 11) and a short follow-up period of 6 months. Because weight reduction after bariatric surgery usually peaks after 24 months, the possible effect of LIRA in poor responders after laparoscopic sleeve gastrectomy may not have been obvious during this short period of administration [116].
Because GLP-1R agonists exert their biological activities by interacting with the GLP-1R, polymorphisms at the GLP-1R gene and interindividual differences in the expression of these receptors may alter biological responses [117]. Early cessation of GLP-1R agonists in individuals unlikely to experience any beneficial metabolic effects can reduce future probable adverse effects, enhance the risk/benefit ratio, and decrease the entire financial cost [118]. Furthermore, the rate of rebound weight gain following the cessation of GLP-1R agonists, as well as the implementation of future strategies to avoid this phenomenon, are other issues that need to be thoroughly addressed in future studies [65]. Recently, the dual GIP/GLP-1 receptor agonist tirzepatide achieved greater body weight reduction than SEMA (at a dose of 1 mg) in patients with T2D [119, 120]. In addition, both dual GLP-1/glucagon receptor agonists and triple GLP-1/GIP/glucagon receptor agonists are under thorough research; their possible effects on individuals with OSA who are overweight or obese should be explored in future studies [121, 122].
SGLT2 inhibitors have also shown encouraging results in individuals with OSA, while individuals with OSA often experience multiple comorbidities that are clinical indications for their administration (Table II). A significant percentage of the lower OSA risk found after their administration was attributable to other pathophysiological mechanisms and not combined changes in traditional risk factors (such as weight loss). Experimental evidence is ongoing to explore and unravel all these possible beneficial mechanisms, while the combination of both drug categories (at least in patients with T2D) with or without other therapeutic strategies seems promising.
Longer, well organized, double-blind RCTs with larger sample sizes in individuals with or without T2D are anticipated in order to investigate the safety, activity, and limitations of both drug categories to decrease OSA severity and improve cardiovascular outcomes. Convincing data with sufficient power to detect the risk/benefit profile of their use remain to be illustrated. This will give valuable information to clinicians to define their future role and adapt targeted therapeutic strategies to give beneficial long-term outcomes. In the meantime, real-world observational studies analysing existing databases can give valuable information for the possible beneficial effects of these 2 drug categories in this challenging, high cardiovascular risk population.